A polilaktinsav (PLA) klasszikus és modern alkalmazásai szövetfeldolgozásban és más orvosbiológiai területeken
Alexandra DREANCĂ1, Amalia Marina NEAGU1, A.V. POTÂRNICHE1, M. BLIDARU2, Codruţa SAROŞI3, Mărioara MOLDOVAN3, I. MARCUS1,*
1 University of Agricultural Sciences and Veterinary Medicine, Faculty of Veterinary Medicine of Cluj-Napoca, Romania
2 "Iuliu Haţieganu" University of Medicine and Pharmacy of Cluj-Napoca, Romania
3 Institute for Research in Chemistry, "Babeș-Bolyai" University of Cluj-Napoca, Romania
* Corresponding author: ioan.marcus@usamvcluj.ro
Abstract
Polylactic acid (PLA) is one of the most investigated biomaterial, being synthesized for the first time by Carothers in 1932 (8). Since then, PLA is produced in large scale all over the globe, its use in medicine being approved by the FDA in 1970 (28). The lactic acid, the PLA substrate is extracted from natural sources, such as feed stock, sugarcane, starch and nowadays from the milk whey (13). Extracting lactic acid from whey and then converting it into polylactic polymer is one of the newly developed strategies for the PLA synthesis (13). Biocompatibility is the property that recommends the PLA as one of the most utilized biopolymer in biomedicine. Due to its physical and mechanical properties, PLA is used in mixtures with other polymers, copolymers, composites or blends (28). The potential applications of the PLA in biomedical field include tissue engineering, wound management, drugs delivery and orthopedics (6). Also, PLA is used to obtain a wide range of products in biomedical fields previously mentioned, such as degradable sutures, drug releasing systems, nano-particles, porous scaffolds, plates, screws for cranio-maxillofacial bone, tacks and pins for ligaments, and nowadays for obtaining soft-tissue implants (6).
The aim of this review paper is to discuss PLAs application in different biomedical fields in order to highlight the PLAs utility as a biomaterial, as well as to enhance the use of composite biomaterials in bone regeneration.
Polylactic acid synthesis
The synthesis of the PLA from lactic acid is made by three polymerization forms, represented by: 1) the condensation for low molecular weight PLA; 2) the ring open polymerization; 3) the chemical catalyzation with high boiling for obtaining PLA with high molecular weight (28). In addition, various studies investigated all the mechanical, physical and chemical properties of the PLA. It is known that the mechanical and crystallization behavior of the PLA is dependent on the molecular weight and stereo chemical makeup of the backbone (8, 16).
Advantages and disadvantages of PLA usage
PLA has 4 advantages in comparison with other biomaterials, which are represented by the renewability, biocompatibility, processability and energy saving (28). Biocompatibility is the most attractive property of PLA with respect to its applications within the biomedical fields. PLA is not toxic in vivo, nor has any carcinogenic effects on the tissues (16). Polylactic polymers degradation occurs by hydrolysis in living organisms tissues, and by thermal activation in nature (16). The products resulting during the PLA degradation are the water and CO , which are non-toxic and further they are 2 metabolized by cells (Fig. 1).
PLA ⇒ Lactic acid ⇒ Piruvic acid ⇒ Krebbs cycle ⇒ CO2 + H2O
Fig.1. Illustration of the chemistry reaction during which the cellular PLA biodegradation occurs
While the PLA has numerous beneficial properties, it also has many drawbacks applications in the medical field. These drawbacks consist in poor toughness and fragility, which makes PLA unsuitable for demanding mechanical performance unless it is suitably modified (5). Another negative aspect is that PLA is hydrophobic, leading to low cell affinity with the appearance of an inflammatory response from the living host tissues (6). PLA has also a slow degradation rate that depends on its molecular weight and crystallinity. Mostly, high molecular weight PLA has a long in vivo life, and the degradation rate lasts from 12 months to 5 years. Another drawback is the lack of the reactive side chain groups, which makes PLAs surface modification a working challenge.
Biomedical applications of the PLA
The tissue engineering synthesizes the substitutes that mimic the natural cell regeneration process to help the growth of new tissue in vitro or in vivo. At a simplistic level, biological tissues consist of cells, signaling mechanisms and cell matrix. An essential condition common to all types of materials used in tissue engineering, especially for scaffolds, is to ensure good biocompatibility (6). PLA is used in bone regeneration mixed with other co-polymers (PLA-PEG or PLA-p-DPEG), or combined with other biological compounds (such as bone morphogenetic proteins-BMPs) for an enhanced tissue regeneration (16). Saito and Takaota (23) examined the use of PLA combined with BMP, and tested their biological effects on severely demineralized bones. Their researches have revealed the bone morphogenetic properties of the mix, but small quantities of bone matter were formed. This disadvantage was influenced by the molecular mass of PLA and its half-life. The study concluded that there was a need for a PLA with a greater molecular weight. Chang et al. (4) created a porous graft from PLA with high molecular weight and BMPs, which achieved a bone matter regeneration effect within two weeks. Hollinger and Schmitz (24) combined demineralized bone with PLA polymers, using the blend in a study on a rabbit with calvarial defect, over 24 weeks. The results have shown that the implant led to the development of a large quantity of trabecular bone matter, without the presence of an inflammatory reaction. Levy et al. (8) used sheets coated with PLA underneath and above the calvarial defect in order to prevent the prolapse of the soft tissue through the defect, and observed an enhanced regeneration process, compared to the control. Agrawal et al. (1) have developed a porous implant of PLA-PGA with added BMPs, and the implants were used in a study on calvarial defects with great bone matter loss. The results have showed a significant increase in bone matter gain in groups with added implants compared to control groups.
Stents
The usage of PLA stents showed lack of inflammatory reaction in the treatment of bladder wall lesions. The advantage of using these stents is that they have degraded in time, while the organ's tissue have regenerated (10). Mixtures between PLA and polytrimetilen carbonate have been tested for prevention of postsurgical adhesions. These biomaterials were used such as films, and proved to be compatible with tissues and non-cytotoxic, preventing postsurgical adhesions (10).
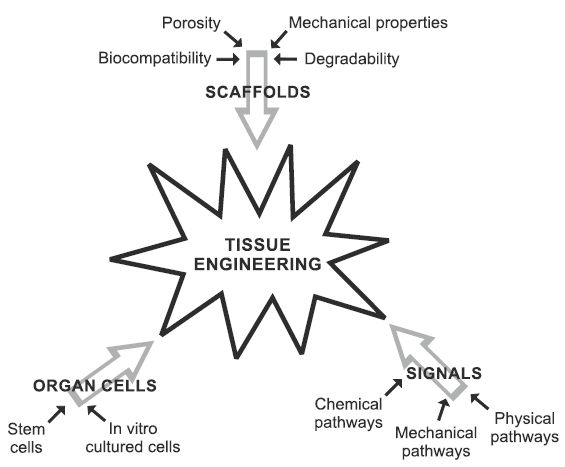
Fig. 2 Required properties and factors important for biomaterials use in the tissue engineering.
The mechanical and accommodation properties of the scaffolds with the host bone tissue must be able to withstand the stress of the daily activity. Tissue engineering technologies are based on a biological triad which involves the successful interaction of 3 components, such as: 1] scaffold that holds the cells to create tissues together in physical form; 2] stem cells that create tissues; and 3] biological signaling molecules, respectively the cellular growth factors (Fig. 2).
Suture material
The first use of PLA for suture materials was reported in 1974. Combined with poliglicolic acid (PGA), the PLA was used for patenting the Vicryl absorbable suture (20). Brekke et al. (3) have tested a suture material composed entirely of the PLA, after the extraction of a molar, and noticed an enhancement of the healing process of the surgical wound, compared to other non-absorbable suture materials used in the dental surgery.
Orthopedics
Biodegradable polymers are used in orthopedics with the purpose of discarding the 2nd surgical intervention for the removal of the medical devices (6, 8). The most commonly used material in orthopedics is titanium, but in the majority of cases it needs to be removed due to the immense mechanical forces it exercises upon the bones or the affected area. In many cases, secondary rejection reactions of titanium by the body are present in animals, as well as in humans, leading to osteomyelitis, bone necrosis, or substance loss over great areas (20). Polylactic acids are used especially in the fabrication of the plates, screws or cerclage wires (2). These materials are used in regions of the body where there is no need for a large mechanical force or toughness, these regions being the joints of the fore- or hind limbs, palmar or plantar faces, and zygomatic bones. Research is on course in rods and screws used in the fixation of long bone fractures, and it consists in the improvement of the mechanical properties, such as impact force, resistance and bending point of PLAs used in surgical procedures on long bones (28). Mending the articular cartilage represents one of the greatest challenges in orthopedics. Schroeder et al. (25) used a PLA matrix with periosteal grafts in the knee of a rabbit. Athanasiou et al. (2) used implants consisting of PLA-PGA combined with growth factors in osteochondral defects in the knee of a rabbit, in order to test the regeneration effect in the cartilage and surrounding bone.
Medical transport systems
Polylactic polymers are used as drug delivery systems (28). These were studied for late-release of varied pharmacological molecules. Discharge of drugs enclosed in polymers is achieved through erosion.Ling and Huang (12) studied the discharge time of the PLA nanoparticles combined with the drug paclitaxel in vitro, where the late discharge of the drug was demonstrated over 6 months. Rancan et al. (21) have studied the utility of the PLA nanoparticles loaded with the fluorescent dye, within the trans-epidermal transport of medicinal substances. The study was carried out on human skin, and revealed the capacity of the PLA to migrate in the hair follicles and to release the pharmacological substances at their base (21).
Testing of PLA on animal bone regeneration models
(Table 1). The bone has the capacity of selfregeneration, but because of certain chronic metabolic pathologies, traumas and aging, deficient bone regeneration occurs (11). Current strategies aim for an enhanced bone tissue healing through implantation of some different biomolecules, such as stem cells, biomaterials and combinations of cell and biomaterials, for the enhancement of the healing process (17).
Testing these compounds on animal models bone defect represents the gold standard in translational research. The goal of biomaterials is to repair, support, substitute or regenerate the wounded bone tissue (17). Relatively few orthopedic experimental models on animals exist in bone engineering, but they allow the most relevant and correct clinical evaluation in the testing of biomaterials. To correct deficiencies, several species are used, such as rats, hares, swine or small ruminants, and the defects concern femur, spine, jaw and calvarial bone (11).
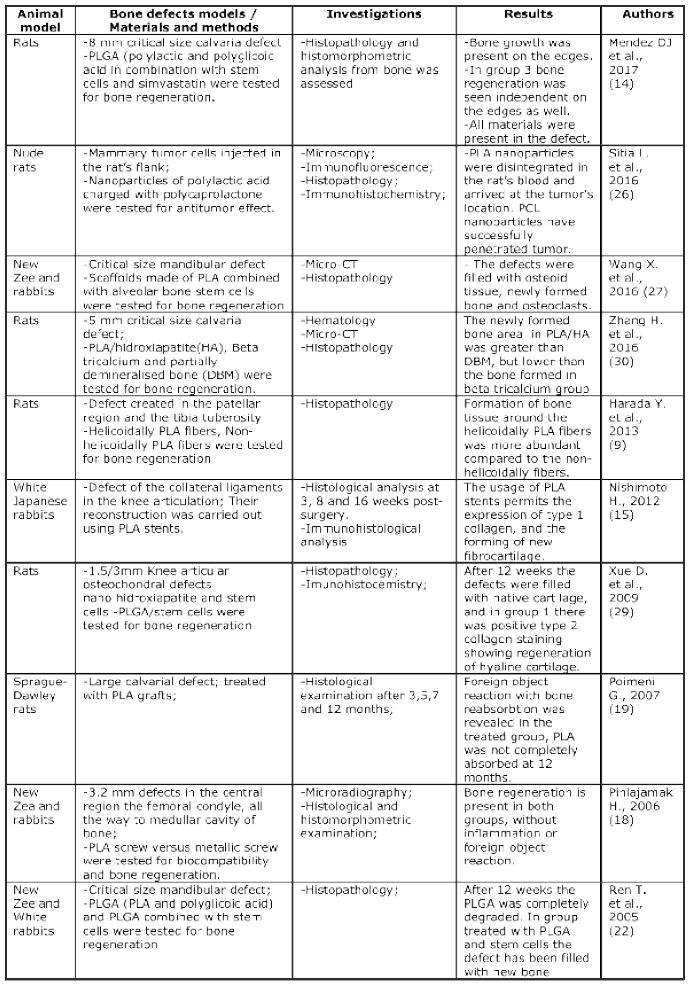
Table 1 Polylactic Acid utilisation in animal bone defect models
Conclusions
- Polylactic acid (PLA) is one of the most studied and utilized biomaterial for the design and clinical use of the biodegradable implants in different scientific biomedical domains.
- PLA is environmental friendly, economical and produced from non-toxic renewable sources.
- PLA is a bone inductive material, used as a leading candidate in the tissue engineering.
- PLA and its copolymers have been tailored for various biomedical and pharmacological purposes and are utilized in numerous patented inventions.
Aknowledgments
This work was supported by a Grant of the Romanian National Authority for Scientific Research and Innovation, CNCS/CCCDI-UEFISCDI, project number PN-III-P2-2.1-BG-2016-0335, within PNCDI III.
References
- Agrawal C.M., (1996), Protein release kinetics of a porous coated implant impregnated with a biodegradable delivery system, Trans Annu Meet Soc Biomater Symp, 2:151
- Athanasiou K.A., (1996), Sterilization, toxicity, biocompatibility and clinical applications of polylactic acid/polyglycolic acid copolymers, Biomaterials, 17:93-102
- Brekke J.H., Olson R.A.J., Scully J.R., Osbon D.B., (1983), Influence of polylactic acid mesh on the incidence of lacalized osteitis, Oral Surgery, Oral Medicine, Oral Pathology, 56:240-245
- Chang P.C., Liu B.Y., Liu C.M., Chou H.H., Ho M.H., Liu H.C., Wang D.M., Hou L.T., (2007), Bone tissue engineering with novel rhBMP2-PLLA composite scaffolds, J Biomed Mater Res A, 81(4):771-780
- Drumright R.E., Gruber P.R., Henton D.E., (2000), Polylactic Acid technology, Advanced Materials, 12(23):1841-1846
- Farah S., Anderson D.G., Langer R., (2016), Physical and mechanical properties of PLA, and their functions in widespread applications - A comprehensive review. Adv Drug Deliv Rev, 107:367-392
- Garlotta D., (2001), A Literature Review of Poly(Lactic Acid), Journal of Polymers and the Environment, 9(2):63-84
- Hamad K.I., Ko Y.G., (2015), Properties and medical applications of polylactic acid: A review, Xpress Polymer Letters, 9(5):435-455
- Harada Y., Kanodo K., Terao T., Suzuki M., Tomita N., (2013), Helical conformation, endows poly-llactic acid fibers with a pizoelectric charge under tensile stress, J Vet Med Sci, 75(9)
- Li G., Wang Z.X., Fu W.J., Hong B.F., Wang X.X., Cao L., Xu F.Q., Song Q., Cui F.Z., Zhang X, (2011), Introduction to biodegradable polylactic acid ureteral stent application for treatment of ureteral war injury, BJU Int, 108(6):901-906
- Li Y., (2015), Bone defect animal models for testing efficacy of bone substitute biomaterials, Journal of Orthopaedic Translation, 3(3):95-104
- Ling Y., Huang Y., (2008), Preparation and Release Efficency of Poly (lactic-co-glycolic) Acid Nanoparticles for Drug Loaded Paclitaxel. In: 7th Asian-Pacific Conference on Medical and Biological Engineering, IFMBE Proceedings, vol 19, (Eds) Y. Peng and X. Weng, Springer, Berlin, Heidelberg, Germany, 514-517
- Maslanka S., Siolek M., Hamryszak L., Lopot D., (2014), Using milk industry waste in production of biodegradable polymers. Chemik, 68(8):703-709
- Mendes Junior D., Domingues J.A., Hausen M.A., Cattani S.M.M., Aragones A., Oliveira A.L.R., Inácio R.F., Barbo M.L.P., Duek E.A.R., (2017), Study of mesenchymal stem cells cultured on a poly(lactic-co-glycolic acid) scaffold containing simvastatin for bone healing. J Appl Biomater Funct Mater, 15(2):e133-e141
- Nishimoto H., Kokubu T., Inui A., Mifune Y., Nishida K., Fujioka H., Yokota K., Hiwa C., Kurosaka M., (2012), Ligament regeneration using an absorbable stent-shaped poly-L-lactic acid scaffold in a rabbit model. Int Orthop, 36(11): 2379-2386
- Pawar R.P., Tekale S.U., Shisodia S.U., Jalinder T.T., Domb A., (2014), Biomedical Applications of Poly(Lactic Acid). Recent Patents on Regenerative Medicine, 4(1):40-51
- Pearce A., Richards R.G., Milz S., Schneider E., Pearce S.G., (2007), Animal models for implant biomaterial research in bone: a review, Eur Cell Mater, 13:1-10.
- Pihlajamäki H., Böstman O., Tynninen O., Laitinen O., (2006), Long-term tissue response to bioabsorbable poly-L-lactide and metallic screws: an experimental study. Bone, 39(4):932-937
- Polimeni G., Koo K.T., Pringle G.A., Agelan A., Safadi F.F., Wikesjö U.M., (2007), Histopathological observations of a Polylactic Acid-Based Device intended for guided bone/tissue regeneration. Clin Implant Dent Relat Res, 10(2):99-105
- Rajendran T., Venugopalan S., (2015), Role of polylactic acid in bone regeneration - A systematic review. J Pharm Sci & Res, 7(11):960-966
- Rancan F., Todorova A., Hadam S., Papakostas D., Luciani E., Graf C., Gernert U., Ruhl E., Verrier B., Sterry W., Vogt A., (2012), Stability of polylactic acid particles and release of fluorochromes upon topical application on human skin explants. Eur J Pharm Biopharm, 80(1):76-84
- Ren T., Ren J., Jia X., Pan K., (2005), The bone formation in vitro and mandibular defect repair using PLGA porous scaffolds. J Biomed Mater Res A, 74(4):562-569
- Saito N., Takaoka K., (2003), New synthetic biodegradable polymers as BMP carriers for bone tissue engineering. Biomaterials, 24(13):2287-2293
- Schmitz J.P., Hollinger J.O., (1988), A preliminary study of the osteogenic potential of biodegradable alloplastic-osteoinductive alloimplant. Clin Orthop Relat Res, 237:245-255
- von Schroeder H.P., Kwan M., Amiel D., Coutts R.D., (1991), The use of polylactic acid matrix and periosteal grafts for the reconstruction of rabbit knee articular defects. J Biomed Mater Res, 25(3): 329-339
- Sitia L., Ferrari R., Violatto M.B., Talamini L., Lupi M., Ubezio P., D'Incalci M., Morbidelli M., Salmona M., Moscatelli D., Bigini P., (2016), Fate of PLA and PCL-based Polymeric Nanoccariers in Cellular and Animal Models of triple-Negative Breast Cancer. Biomacromolecules, 17(3):744-755
- Wang X., Xing H., Zhang G., Wu X., Wang D., Li M., Zhao J., Du X., Lv Y., Lingling E., Liu H., (2016), Restoration of a Critical Mandibular Bone defect using Human alveolar bone-derived stem cells and porous Nano-HA/Collagen/PLA Scaffold. Stem Cells International, 2016:1-13
- Xiao L., Wang B., Yang G., Guthier M., (2012), Poly(Lactic Acid) - Based Biomaterials: Synthesis, Modification and Applications. Biomedical Science, Engineering and technology, Intech Open Access, 11:248-268
- Xue D., Zheng Q., Zong C., Li Q., Li H., Qian S., Zhang B., Yu L., Pan Z., (2009), Osteochondral repair using porous poly(lactide-co-glicolide)/nano- hydroxiapatite hybrid scaffolds with undifferentiated mesenchymal stem cells in a rat model. J Biomed Mater Res A, 94(1):259-270
- Zhang H., Mao X., Du Z., Jiang W., Han X., Zhao D., Han D., Li Q., (2016), Three dimensional printed macroporous polylactic acid/hidroxyapatite composite scaffols for promoting bone formation in a critical-size rat calvarial defect model. Sci Technol Adv Mater, 17(1):136-148